The aufbau process is a set of rules that allows us to predict the electronic configuration of an atom if we know how many electrons there are in the atom. If the periodic table is used as a tool, this process is pretty easy.
Periodic Table Explorer是一款元素周期表软件,它在普通元素周期表的基础上,为每种元素都配备了图片。 适合儿童学习。 爱奇艺 腾讯视频 鲜牛加速器 金山毒霸 雷神加速器7.0 2345浏览器. Interactive periodic table with up-to-date element property data collected from authoritative sources. Look up chemical element names, symbols, atomic masses and other properties, visualize trends, or even test your elements knowledge by playing a periodic table game!
For atoms found in the first two columns of the periodic table (figure AT5.1), the configuration is a closed shell of core electrons, plus s electrons in a new shell. For example, potassium has a configuration [Ar]4s1. These atoms are often called the alkali and alkaline earth elements. Alkali elements, from the first column, have a configuration ending in s1; alkaline earth elements, from the second column, have configurations ending in s2. Together, these elements are often called the s-block elements, because their valence electrons are s electrons. Remember, the valence electrons are the ones beyond the noble gas core. In the case of potassium, they are the ones beyond [Ar].
Figure AT6.1. The periodic table. Red elements are the alkali and alkaline earth metals (s-block). Yellow elements are the transition metals (d-block). Orange elements are the lanthanides and actinides (f-block). Green, blue and purple elements are the p-block; together with the s-block, they are called the main group elements. The main group is divided into metals (green), metalloids (teal), non-metals (blue) and noble or inert gases (purple).
The first two and the last six columns of the periodic table are called the main group elements. Alternatively, they are sometimes called the s-block and p-block elements, respectively. For example, phosphorus has a configuration, [Ne]4s24px1py1pz1, or simply [Ne]4s24p3.
The middle block of the periodic table consists of the transition metals or the d-block elements. For example, scandium has configuration [Ne]4s23d1.
The final two rows of the periodic table are the lanthanides and actinides. Collectively, they are called the f-block elements. Samarium, for example, is [Xe]6s24f6. These elements could really be inserted at the left-hand side of the d-block in the appropriate rows. Notice that lanthanum, element 57, is followed by hafnium, element 72, in the table. The element that really occurs next is element 58, cerium, and it is shown in the lanthanide row down below. The f-block elements are usually shown below in order to save space.
Really, the periodic table should look like this:
Figure AT6.2. The periodic table shown with the lanthanides in their proper places.
- The periodic table is divided into columns of atoms with similar electron configurations.
- Atoms with similar electron configurations have similar properties.
Chemical reactions depend on the movement of electrons. In a reaction, one atom may accept electrons from another atom. One atom may donate electrons to another atoms. The valence electrons are the outermost electrons in an atom; they are closest to the surface of an atom. That fact makes the valence electrons more likely to interact with other atoms. The valence are also the highest-energy electrons in an atom, and most likely to participate in a reaction.
For these reasons, atoms with similar electron configurations generally behave in similar ways. The repeating properties in each row of the periodic table, as observed by Mendeleev and others, reflect the repeating electron configurations in subsequent rows. The periodic table organizes atoms with similar configurations and properties together in columns.
Problem AT6.1.
For the following elements, suggest two other elements that would have similar properties.
a) zinc, Zn b) calcium, Ca c) oxygen, O d) chlorine, Cl e) chromium, Cr
Problem AT6.2.
Make a diagram showing the energy levels of different orbitals, arranged by principal quantum number.
'Periodic trends' refer to the way in which physical properties of atoms change across the periodic table. One of the most commonly used periodic trends in chemistry is electronegativity. Electronegativity is closely connected to the basic idea of chemical reactions: the transfer of an electron from one neutral atom to another. It refers to how strongly an atom attracts electrons from other atoms.
- Electronegativity is a measure of an atom's ability to draw electrons towards itself, or the ability of the nucleus to hold electrons tightly.
There are many scales of electronegativity, based on different physical measurements. Usually, electronegativity is set to an approximately 4-point scale. Atoms with electronegativity of around 4 draw electrons very strongly toward themselves. Atoms with electronegativity of 1 (or lower) only weakly draw electrons toward themselves.
The following data use the Allen scale of electronegativity. The Allen scale uses spectroscopic measurements to estimate the energy of valence electrons in an atom. From these values, the relative attraction of the atom for its valence electrons is placed on a 4 point scale (approximately).
Table AT6.1. The Allen electronegativity values of the second-row elements.
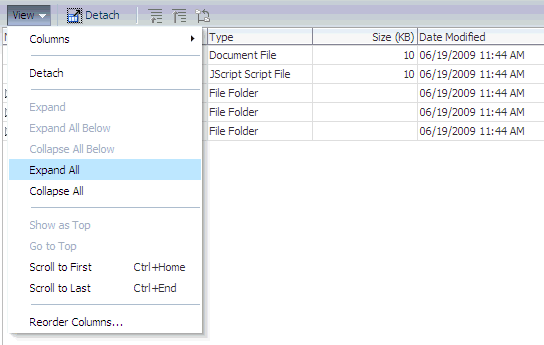
Some electronegativity scales do not have values for the noble gases, because they are based on experimental measurements of compounds, and noble gases do not commonly form compounds with other elements. Instead, they exist as single atoms. The Allen scale just depends on the ability of an atom to interact with light, which is something even noble gases can do. As a result, noble gases are also given electronegativity values on this scale. However, on many scales, fluorine would be the most electronegative atom here. As a result, fluorine is usually thought of as the most electronegative element.
Often it is useful to plot data on a graph. That way, we can get a better look at the relationship. For example, a quick glance at Figure AT5.2. shows that there is a smooth increase in electronegativity as we move across a row in the periodic table.
Figure AT6.3. A plot of electronegativity versus atomic number in the second row of the periodic table.
Problem AT6.3.
Take a look at the graph in figure AT6.2. Can you explain why the electronegativity increases as atomic number increases?.
Problem AT6.4.
Suppose you need an electron. You have a boron atom and an oxygen atom. You try to take an electron away from one. Use Figure AT6.2. to predict which atom will give up the electron more easily.
Problem AT6.5.
Suppose you have an electron. You are able to send it into a vessel that contains a carbon atom and a fluorine atom. Use Figure AT6.2. to predict which atom is more likely to take the electron.
Problem AT6.6.
A covalent chemical bond is a pair of electrons shared between two atoms. Suppose you have a carbon-oxygen bond. Will the electrons be shared evenly between the two atoms, or will one atom pull the electrons more tightly towards itself? Use Figure AT6.2. to make your prediction.
What is happening as we move across a row in the periodic table? Why does electronegativity increase?
Keep in mind that the only difference from one element to the next is the number of protons in the nucleus. The number of protons is called the atomic number. If you know the number of protons you have, then you know what atoms you have. Electronegativity may have something to do with the number of protons in the nucleus. In fact, it should. The more protons there are in the nucleus, the more strongly electrons should be attracted to it. Each additional proton should add more electrostatic attraction for an electron. Fluorine, with nine protons, should attract electrons much more strongly than lithium, which has only three protons.
- Moving across a row of the periodic table, as protons are added to the nucleus, electrons are held more tightly.
- Electronegativity increases across a row in the periodic table.
It seems like that effect should be offset by the increasing number of electrons in the atom. Each time a proton is added, so is an electron. That electron should repel other electrons in the atom, cancelling out the effect of more protons in the nucleus.
However, the structure of the atom minimizes electron-electron repulsion a little bit. Remember that all the protons are in one place, the nucleus. All the electrons are a relatively great distance from the nucleus, in many different directions. Chances are, an additional electron is much farther away; it may be twice as far away as an additional proton in the nucleus. It may be all the way on the other side of the atom. Because electrons are spread out in the atom, and the distances between them is pretty large, the repulsive effect is a little smaller than the attractive effect of additional protons.
We can see that this trend is generally true across the periodic table, with a few exceptions here and there.
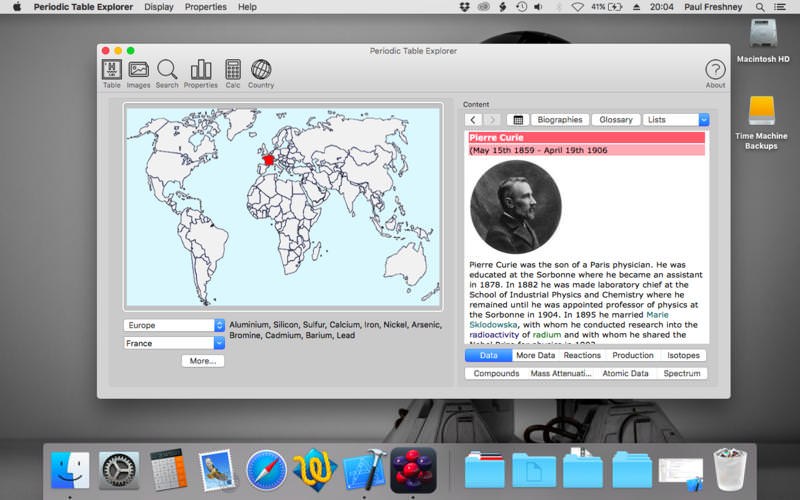
Figure AT6.4. Electronegativity trends across the periodic table. Download a copy.
What happens as we move down a column in the periodic table? Table AT5.2. shows the Allen electronegativities of the alkali metals. These elements are also called the Group 1 elements or the Group IA elements. The 'Group 1' designation is used because they are the first column or group in the periodic table. The data are also presented in Figure AT5.4.
Table AT6.2. The Allen electronegativity values of the alkali elements.
Figure AT6.5. Plot of the Allen electronegativity values of the alkali elements.

There is a different trend here. In this case, lithium (atomic number 3) has more protons than hydrogen (atomic number 1). However, hydrogen is a lot more electronegative than lithium. Francium, with 87 protons in its nucleus, is the least electronegative alkali element.
Problem AT6.7.
Take a look at the graph in figure AT6.4. Can you explain why the electronegativity decreases as atomic number increases, going down this column?
Problem AT6.8.
An ionic chemical bond is a pair of ions attracted by their opposite charges. A cation is a positively charged ion; it may be an atom that has lost an electron. An anion is a negatively charged ion; it may be an atom that has gained an extra electron. Ions can form by moving an electron from one atom to another.
a) Suppose you have an ionic cesium-fluorine bond. Which ion is the cesium and which is the fluorine? Use Figure AT6.2 and Figure AT6.4. to make your prediction. Cesium is Cs.
b) Suppose you have an ionic sodium-oxygen bond. Which ion is the sodium and which is the oxygen? Use Figure AT6.2 and Figure AT6.4. to make your prediction. Sodium is Na (from the Latin, natrium).
c) Suppose you have an ionic potassium-hydrogen bond. Which ion is the potassium and which is the hydrogen? Use Figure AT6.2 and Figure AT6.4. to make your prediction. Potassium is K (from the Latin, kalium).
Periodic Trends and Atomic Radius
The biggest difference between two atoms in the same group (column) in the periodic table is the principal quantum number. Remember, that corresponds to the 'valence shell'. Think of electrons as forming layers around the nucleus. Electrons with principal quantum number one form a first layer. Those with principal quantum number 2 form a second layer, and so on. Each layer is further away from the nucleus. Remember, electrostatic attraction gets weaker as charges get further away from each other. As electrons get further from the nucleus, they are less tightly held.
- Moving down a column in the periodic table, valence electrons are held less tightly because they get further from the nucleus.
- Electronegativity decreases as we move down a column in the periodic table.
We can see this general size trend in the following periodic table. This table presents covalent radii, which are related to the sizes of the atoms (although not exactly the same; data on atomic radii are not available for all atoms, however).
Figure AT6.6. Covalent radii of the atoms.Download a copy here.
We can clearly see the expanding radii of atoms if we look at Group 1, the first column; these elements are called the alkali metals. Hydrogen, at the top, is very small. Lithium is much bigger. Sodium is much bigger than lithium, however, and potassium is much bigger than sodium. And so on: francium is bigger than cesium, which is bigger than rubidium, which is bigger than potassium.
Each time an electron is added to an orbital that is significantly farther from the nucleus, of course it is going to result in a bigger atom. Remember, the atom is mostly empty space, and its size is described by the outermost reaches of its electrons. So when we go to the next principal quantum number -- that is, to the next row in the periodic table, from the first row to the second row, for example -- the next electron is much further away from the nucleus. It has to be that way, because electrons repel each other. They can't all be equally close to the nucleus, because there would be too much repulsion. Instead, they form these layers, and when the first layer is so full that there would be too much repulsion if anothe relectron were added, we start the next layer.
Of course, the very first layer is very, very small. There just isn't that much room so close to the nucleus. For the first row, only two electrons are allowed. Then they have to start the next layer. For the second row, eight electrons are allowed; that's the origin of something called the 'octet rule' (think 'octopus') for common compounds, which you'll see later on. Eventually we get to eighteen electrons in a shell, then thirty two, as the shells get bigger and bigger like layers of an onion, or like nested Russian dolls.
There is another important trend if you look carefully. As you move from left to right across the periodic table, from one group to the next, the atoms get bigger. That doesn't make any sense, does it? If we are adding more electrons, why would the atom get smaller?
The key thing is, not only are we adding more electrons, but we are also adding more protons in the nucleus. The new electrons we are adding are all roughly equidistant from the nucleus; they are all equally close to the protons. So as the charge on the nucleus gets bigger, those electrons are all more strongly attracted to the centre. The atom shrinks.
Eventually, we get to the point at which we couldn't possibly add more electrons; the radius has shrunk so much that repulsion would become too great if we added one more electron. Then we just start another row. Just before that point, however, we hit a sweet spot: the point at which the attraction between the nucleus and the outermost electrons is so strong, and the electrons are held so tightly, that the atom becomes very, very stable. This last column in the table contains the noble gases, which are particularly stable and unreactive.
Problem AT6.9.
Why does electronegativity fall so sharply between hydrogen and lithium, and much more subtly between lithium and sodium?
Problem AT6.10.
Which atom, in the following pairs, is more electronegative?
a) magnesium, Mg, or calcium, Ca
b) lead, Pb, or tin, Sn
c) silver, Ag, or antimony, Sb
d) gallium, Ga, or arsenic, As
e) tungsten, W, or copper, Cu
Periodic Table Explorer 1.6 4.0
f) thallium, Tl, or sulfur, S
Problem AT6.11.
Electron ionization is the energy that must be added in order to pull an electron away from an atom.
a) Why do you think energy has to be used to pull an electron away from an atom? What is holding the electron there?
b) Explain the general trend in ionization energies seen in the following table (a larger value means more energy must be added to remove a first electron from the atom).
Table AT6.6. The ionization energies of the second row elements.
Problem AT6.12.
Sometimes a plot of the data can be revealing. Ionization energies do not follow a smooth trend. Explain why it is a little easier to remove an electron from boron and oxygen than expected. (Electron configurations may be helpful here.)
Figure AT6.7. Plot of the ionization energies of the second row elements.
Problem AT6.13.
Explain the trend in the following data on ionization energy.
Table AT6.4. The ionization energies of the alkali elements.
Problem AT6.14.
Electron affinity is the energy released when a free electron is picked up by an atom.
a) Why would energy be released when a free electron is taken by an atom?
b) Explain the general trend in the following electron affinity data.
Table AT6.5. The electron affinities of the alkali elements.
Problem AT6.15.
a) Explain a general trend in the following electron affinity data.
b) There are several exceptions to the general trend. Why do beryllium and neon have such low electron affinities (almost zero)?
c) Nitrogen also has an electron affinity that is close to zero. Why?
Problem AT6.16.
Usually, elements become bigger as we go down a column in the periodic table. However, in a phenomenon called 'the lanthanide contraction', some elements are actually smaller than the ones in the row above them. Specifically, osmium, iridium, platinum, gold, and mercury are smaller than their relatives, ruthenium, rhodium, palladium, silver, and cadmium, respectively.
Use the periodic table in Figure AT6.2. to offer a possible explanation for this phenomenon.
Click a column title, such as Name, to sort the table by that item.
SEENotes at the bottom of the Table.
No. | Atomic Weight | Name | Sym. | M.P. (°C) | B.P. (°C) | Density* (g/cm3) | Earth crust (%)* | Discovery (Year) | Group* | Electron configuration | Ionization energy (eV) | |
---|---|---|---|---|---|---|---|---|---|---|---|---|
1 | 1.008 | Hydrogen | H | -259 | -253 | 0.09 | 0.14 | 1776 | 1 | 1s1 | 13.60 | |
2 | 4.003 | Helium | He | -272 | -269 | 0.18 | 1895 | 18 | 1s2 | 24.59 | ||
3 | 6.941 | Lithium | Li | 180 | 1,347 | 0.53 | 1817 | 1 | [He] 2s1 | 5.39 | ||
4 | 9.012 | Beryllium | Be | 1,278 | 2,970 | 1.85 | 1797 | 2 | [He] 2s2 | 9.32 | ||
5 | 10.811 | Boron | B | 2,300 | 2,550 | 2.34 | 1808 | 13 | [He] 2s2 2p1 | 8.30 | ||
6 | 12.011 | Carbon | C | 3,500 | 4,827 | 2.26 | 0.09 | ancient | 14 | [He] 2s2 2p2 | 11.26 | |
7 | 14.007 | Nitrogen | N | -210 | -196 | 1.25 | 1772 | 15 | [He] 2s2 2p3 | 14.53 | ||
8 | 15.999 | Oxygen | O | -218 | -183 | 1.43 | 46.71 | 1774 | 16 | [He] 2s2 2p4 | 13.62 | |
9 | 18.998 | Fluorine | F | -220 | -188 | 1.70 | 0.03 | 1886 | 17 | [He] 2s2 2p5 | 17.42 | |
10 | 20.180 | Neon | Ne | -249 | -246 | 0.90 | 1898 | 18 | [He] 2s2 2p6 | 21.56 | ||
11 | 22.990 | Sodium | Na | 98 | 883 | 0.97 | 2.75 | 1807 | 1 | [Ne] 3s1 | 5.14 | |
12 | 24.305 | Magnesium | Mg | 639 | 1,090 | 1.74 | 2.08 | 1755 | 2 | [Ne] 3s2 | 7.65 | |
13 | 26.982 | Aluminum | Al | 660 | 2,467 | 2.70 | 8.07 | 1825 | 13 | [Ne] 3s2 3p1 | 5.99 | |
14 | 28.086 | Silicon | Si | 1,410 | 2,355 | 2.33 | 27.69 | 1824 | 14 | [Ne] 3s2 3p2 | 8.15 | |
15 | 30.974 | Phosphorus | P | 44 | 280 | 1.82 | 0.13 | 1669 | 15 | [Ne] 3s2 3p3 | 10.49 | |
16 | 32.065 | Sulfur | S | 113 | 445 | 2.07 | 0.05 | ancient | 16 | [Ne] 3s2 3p4 | 10.36 | |
17 | 35.453 | Chlorine | Cl | -101 | -35 | 3.21 | 0.05 | 1774 | 17 | [Ne] 3s2 3p5 | 12.97 | |
18 | 39.948 | Argon | Ar | -189 | -186 | 1.78 | 1894 | 18 | [Ne] 3s2 3p6 | 15.76 | ||
19 | 39.098 | Potassium | K | 64 | 774 | 0.86 | 2.58 | 1807 | 1 | [Ar] 4s1 | 4.34 | |
20 | 40.078 | Calcium | Ca | 839 | 1,484 | 1.55 | 3.65 | 1808 | 2 | [Ar] 4s2 | 6.11 | |
21 | 44.956 | Scandium | Sc | 1,539 | 2,832 | 2.99 | 1879 | 3 | [Ar] 3d1 4s2 | 6.56 | ||
22 | 47.867 | Titanium | Ti | 1,660 | 3,287 | 4.54 | 0.62 | 1791 | 4 | [Ar] 3d2 4s2 | 6.83 | |
23 | 50.942 | Vanadium | V | 1,890 | 3,380 | 6.11 | 1830 | 5 | [Ar] 3d3 4s2 | 6.75 | ||
24 | 51.996 | Chromium | Cr | 1,857 | 2,672 | 7.19 | 0.04 | 1797 | 6 | [Ar] 3d5 4s1 | 6.77 | |
25 | 54.938 | Manganese | Mn | 1,245 | 1,962 | 7.43 | 0.09 | 1774 | 7 | [Ar] 3d5 4s2 | 7.43 | |
26 | 55.845 | Iron | Fe | 1,535 | 2,750 | 7.87 | 5.05 | ancient | 8 | [Ar] 3d6 4s2 | 7.90 | |
27 | 58.933 | Cobalt | Co | 1,495 | 2,870 | 8.90 | 1735 | 9 | [Ar] 3d7 4s2 | 7.88 | ||
28 | 58.693 | Nickel | Ni | 1,453 | 2,732 | 8.90 | 0.02 | 1751 | 10 | [Ar] 3d8 4s2 | 7.64 | |
29 | 63.546 | Copper | Cu | 1,083 | 2,567 | 8.96 | ancient | 11 | [Ar] 3d10 4s1 | 7.73 | ||
30 | 65.390 | Zinc | Zn | 420 | 907 | 7.13 | ancient | 12 | [Ar] 3d10 4s2 | 9.39 | ||
31 | 69.723 | Gallium | Ga | 30 | 2,403 | 5.91 | 1875 | 13 | [Ar] 3d10 4s2 4p1 | 6.00 | ||
32 | 72.640 | Germanium | Ge | 937 | 2,830 | 5.32 | 1886 | 14 | [Ar] 3d10 4s2 4p2 | 7.90 | ||
33 | 74.922 | Arsenic | As | 81 | 613 | 5.72 | ancient | 15 | [Ar] 3d10 4s2 4p3 | 9.79 | ||
34 | 78.960 | Selenium | Se | 217 | 685 | 4.79 | 1817 | 16 | [Ar] 3d10 4s2 4p4 | 9.75 | ||
35 | 79.904 | Bromine | Br | -7 | 59 | 3.12 | 1826 | 17 | [Ar] 3d10 4s2 4p5 | 11.81 | ||
36 | 83.800 | Krypton | Kr | -157 | -153 | 3.75 | 1898 | 18 | [Ar] 3d10 4s2 4p6 | 14.00 | ||
37 | 85.468 | Rubidium | Rb | 39 | 688 | 1.63 | 1861 | 1 | [Kr] 5s1 | 4.18 | ||
38 | 87.620 | Strontium | Sr | 769 | 1,384 | 2.54 | 1790 | 2 | [Kr] 5s2 | 5.69 | ||
39 | 88.906 | Yttrium | Y | 1,523 | 3,337 | 4.47 | 1794 | 3 | [Kr] 4d1 5s2 | 6.22 | ||
40 | 91.224 | Zirconium | Zr | 1,852 | 4,377 | 6.51 | 0.03 | 1789 | 4 | [Kr] 4d2 5s2 | 6.63 | |
41 | 92.906 | Niobium | Nb | 2,468 | 4,927 | 8.57 | 1801 | 5 | [Kr] 4d4 5s1 | 6.76 | ||
42 | 95.940 | Molybdenum | Mo | 2,617 | 4,612 | 10.22 | 1781 | 6 | [Kr] 4d5 5s1 | 7.09 | ||
43 | * | 98.000 | Technetium | Tc | 2,200 | 4,877 | 11.50 | 1937 | 7 | [Kr] 4d5 5s2 | 7.28 | |
44 | 101.070 | Ruthenium | Ru | 2,250 | 3,900 | 12.37 | 1844 | 8 | [Kr] 4d7 5s1 | 7.36 | ||
45 | 102.906 | Rhodium | Rh | 1,966 | 3,727 | 12.41 | 1803 | 9 | [Kr] 4d8 5s1 | 7.46 | ||
46 | 106.420 | Palladium | Pd | 1,552 | 2,927 | 12.02 | 1803 | 10 | [Kr] 4d10 | 8.34 | ||
47 | 107.868 | Silver | Ag | 962 | 2,212 | 10.50 | ancient | 11 | [Kr] 4d10 5s1 | 7.58 | ||
48 | 112.411 | Cadmium | Cd | 321 | 765 | 8.65 | 1817 | 12 | [Kr] 4d10 5s2 | 8.99 | ||
49 | 114.818 | Indium | In | 157 | 2,000 | 7.31 | 1863 | 13 | [Kr] 4d10 5s2 5p1 | 5.79 | ||
50 | 118.710 | Tin | Sn | 232 | 2,270 | 7.31 | ancient | 14 | [Kr] 4d10 5s2 5p2 | 7.34 | ||
51 | 121.760 | Antimony | Sb | 630 | 1,750 | 6.68 | ancient | 15 | [Kr] 4d10 5s2 5p3 | 8.61 | ||
52 | 127.600 | Tellurium | Te | 449 | 990 | 6.24 | 1783 | 16 | [Kr] 4d10 5s2 5p4 | 9.01 | ||
53 | 126.905 | Iodine | I | 114 | 184 | 4.93 | 1811 | 17 | [Kr] 4d10 5s2 5p5 | 10.45 | ||
54 | 131.293 | Xenon | Xe | -112 | -108 | 5.90 | 1898 | 18 | [Kr] 4d10 5s2 5p6 | 12.13 | ||
55 | 132.906 | Cesium | Cs | 29 | 678 | 1.87 | 1860 | 1 | [Xe] 6s1 | 3.89 | ||
56 | 137.327 | Barium | Ba | 725 | 1,140 | 3.59 | 0.05 | 1808 | 2 | [Xe] 6s2 | 5.21 | |
57 | 138.906 | Lanthanum | La | 920 | 3,469 | 6.15 | 1839 | 3 | [Xe] 5d1 6s2 | 5.58 | ||
58 | 140.116 | Cerium | Ce | 795 | 3,257 | 6.77 | 1803 | 101 | [Xe] 4f1 5d1 6s2 | 5.54 | ||
59 | 140.908 | Praseodymium | Pr | 935 | 3,127 | 6.77 | 1885 | 101 | [Xe] 4f3 6s2 | 5.47 | ||
60 | 144.240 | Neodymium | Nd | 1,010 | 3,127 | 7.01 | 1885 | 101 | [Xe] 4f4 6s2 | 5.53 | ||
61 | * | 145.000 | Promethium | Pm | 1,100 | 3,000 | 7.30 | 1945 | 101 | [Xe] 4f5 6s2 | 5.58 | |
62 | 150.360 | Samarium | Sm | 1,072 | 1,900 | 7.52 | 1879 | 101 | [Xe] 4f6 6s2 | 5.64 | ||
63 | 151.964 | Europium | Eu | 822 | 1,597 | 5.24 | 1901 | 101 | [Xe] 4f7 6s2 | 5.67 | ||
64 | 157.250 | Gadolinium | Gd | 1,311 | 3,233 | 7.90 | 1880 | 101 | [Xe] 4f7 5d1 6s2 | 6.15 | ||
65 | 158.925 | Terbium | Tb | 1,360 | 3,041 | 8.23 | 1843 | 101 | [Xe] 4f9 6s2 | 5.86 | ||
66 | 162.500 | Dysprosium | Dy | 1,412 | 2,562 | 8.55 | 1886 | 101 | [Xe] 4f10 6s2 | 5.94 | ||
67 | 164.930 | Holmium | Ho | 1,470 | 2,720 | 8.80 | 1867 | 101 | [Xe] 4f11 6s2 | 6.02 | ||
68 | 167.259 | Erbium | Er | 1,522 | 2,510 | 9.07 | 1842 | 101 | [Xe] 4f12 6s2 | 6.11 | ||
69 | 168.934 | Thulium | Tm | 1,545 | 1,727 | 9.32 | 1879 | 101 | [Xe] 4f13 6s2 | 6.18 | ||
70 | 173.040 | Ytterbium | Yb | 824 | 1,466 | 6.90 | 1878 | 101 | [Xe] 4f14 6s2 | 6.25 | ||
71 | 174.967 | Lutetium | Lu | 1,656 | 3,315 | 9.84 | 1907 | 101 | [Xe] 4f14 5d1 6s2 | 5.43 | ||
72 | 178.490 | Hafnium | Hf | 2,150 | 5,400 | 13.31 | 1923 | 4 | [Xe] 4f14 5d2 6s2 | 6.83 | ||
73 | 180.948 | Tantalum | Ta | 2,996 | 5,425 | 16.65 | 1802 | 5 | [Xe] 4f14 5d3 6s2 | 7.55 | ||
74 | 183.840 | Tungsten | W | 3,410 | 5,660 | 19.35 | 1783 | 6 | [Xe] 4f14 5d4 6s2 | 7.86 | ||
75 | 186.207 | Rhenium | Re | 3,180 | 5,627 | 21.04 | 1925 | 7 | [Xe] 4f14 5d5 6s2 | 7.83 | ||
76 | 190.230 | Osmium | Os | 3,045 | 5,027 | 22.60 | 1803 | 8 | [Xe] 4f14 5d6 6s2 | 8.44 | ||
77 | 192.217 | Iridium | Ir | 2,410 | 4,527 | 22.40 | 1803 | 9 | [Xe] 4f14 5d7 6s2 | 8.97 | ||
78 | 195.078 | Platinum | Pt | 1,772 | 3,827 | 21.45 | 1735 | 10 | [Xe] 4f14 5d9 6s1 | 8.96 | ||
79 | 196.967 | Gold | Au | 1,064 | 2,807 | 19.32 | ancient | 11 | [Xe] 4f14 5d10 6s1 | 9.23 | ||
80 | 200.590 | Mercury | Hg | -39 | 357 | 13.55 | ancient | 12 | [Xe] 4f14 5d10 6s2 | 10.44 | ||
81 | 204.383 | Thallium | Tl | 303 | 1,457 | 11.85 | 1861 | 13 | [Xe] 4f14 5d10 6s2 6p1 | 6.11 | ||
82 | 207.200 | Lead | Pb | 327 | 1,740 | 11.35 | ancient | 14 | [Xe] 4f14 5d10 6s2 6p2 | 7.42 | ||
83 | 208.980 | Bismuth | Bi | 271 | 1,560 | 9.75 | ancient | 15 | [Xe] 4f14 5d10 6s2 6p3 | 7.29 | ||
84 | * | 209.000 | Polonium | Po | 254 | 962 | 9.30 | 1898 | 16 | [Xe] 4f14 5d10 6s2 6p4 | 8.42 | |
85 | * | 210.000 | Astatine | At | 302 | 337 | 0.00 | 1940 | 17 | [Xe] 4f14 5d10 6s2 6p5 | 9.30 | |
86 | * | 222.000 | Radon | Rn | -71 | -62 | 9.73 | 1900 | 18 | [Xe] 4f14 5d10 6s2 6p6 | 10.75 | |
87 | * | 223.000 | Francium | Fr | 27 | 677 | 0.00 | 1939 | 1 | [Rn] 7s1 | 4.07 | |
88 | * | 226.000 | Radium | Ra | 700 | 1,737 | 5.50 | 1898 | 2 | [Rn] 7s2 | 5.28 | |
89 | * | 227.000 | Actinium | Ac | 1,050 | 3,200 | 10.07 | 1899 | 3 | [Rn] 6d1 7s2 | 5.17 | |
90 | 232.038 | Thorium | Th | 1,750 | 4,790 | 11.72 | 1829 | 102 | [Rn] 6d2 7s2 | 6.31 | ||
91 | 231.036 | Protactinium | Pa | 1,568 | 0 | 15.40 | 1913 | 102 | [Rn] 5f2 6d1 7s2 | 5.89 | ||
92 | 238.029 | Uranium | U | 1,132 | 3,818 | 18.95 | 1789 | 102 | [Rn] 5f3 6d1 7s2 | 6.19 | ||
93 | * | 237.000 | Neptunium | Np | 640 | 3,902 | 20.20 | 1940 | 102 | [Rn] 5f4 6d1 7s2 | 6.27 | |
94 | * | 244.000 | Plutonium | Pu | 640 | 3,235 | 19.84 | 1940 | 102 | [Rn] 5f6 7s2 | 6.03 | |
95 | * | 243.000 | Americium | Am | 994 | 2,607 | 13.67 | 1944 | 102 | [Rn] 5f7 7s2 | 5.97 | |
96 | * | 247.000 | Curium | Cm | 1,340 | 0 | 13.50 | 1944 | 102 | 5.99 | ||
97 | * | 247.000 | Berkelium | Bk | 986 | 0 | 14.78 | 1949 | 102 | 6.20 | ||
98 | * | 251.000 | Californium | Cf | 900 | 0 | 15.10 | 1950 | 102 | 6.28 | ||
99 | * | 252.000 | Einsteinium | Es | 860 | 0 | 0.00 | 1952 | 102 | 6.42 | ||
100 | * | 257.000 | Fermium | Fm | 1,527 | 0 | 0.00 | 1952 | 102 | 6.50 | ||
101 | * | 258.000 | Mendelevium | Md | 0 | 0 | 0.00 | 1955 | 102 | 6.58 | ||
102 | * | 259.000 | Nobelium | No | 827 | 0 | 0.00 | 1958 | 102 | 6.65 | ||
103 | * | 262.000 | Lawrencium | Lr | 1,627 | 0 | 0.00 | 1961 | 102 | 4.90 | ||
104 | * | 261.000 | Rutherfordium | Rf | 0 | 0 | 0.00 | 1964 | 4 | 0.00 | ||
105 | * | 262.000 | Dubnium | Db | 0 | 0 | 0.00 | 1967 | 5 | 0.00 | ||
106 | * | 266.000 | Seaborgium | Sg | 0 | 0 | 0.00 | 1974 | 6 | 0.00 | ||
107 | * | 264.000 | Bohrium | Bh | 0 | 0 | 0.00 | 1981 | 7 | 0.00 | ||
108 | * | 277.000 | Hassium | Hs | 0 | 0 | 0.00 | 1984 | 8 | 0.00 | ||
109 | * | 268.000 | Meitnerium | Mt | 0 | 0 | 0.00 | 1982 | 9 | 0.00 | ||
No. | Atomic Weight | Name | Sym. | M.P. (°C) | B.P. (°C) | Density* (g/cm3) | Earth crust (%)* | Discovery (Year) | Group* | Electron configuration | Ionization energy (eV) |
Notes:
• Density of elements with boiling points below 0°C is given in g/l. In a sorted list these elements are shown before other elements that have boiling points >0°C.
• Earth crust composition average values are from a report by F. W. Clarke and H. S. Washington, 1924. Elemental composition of crustal rocks differ between different localities (see article).
• Group: There are only 18 groups in the periodic table that constitute the columns of the table. Lanthanoids and Actinoids are numbered as 101 and 102 to separate them in sorting by group.
• The elements marked with an asterisk (in the 2nd column) have no stable nuclides. For these elements the weight value shown represents the mass number of the longest-lived isotope of the element.
Abbreviations and Definitions:
No. - Atomic Number
M.P. - melting point
B.P. - boiling point
Atomic number: The number of protons in an atom. Each element is uniquely defined by its atomic number.
Atomic mass: The mass of an atom is primarily determined by the number of protons and neutrons in its nucleus. Atomic mass is measured in Atomic Mass Units (amu) which are scaled relative to carbon, 12C, that is taken as a standard element with an atomic mass of 12. This isotope of carbon has 6 protons and 6 neutrons. Thus, each proton and neutron has a mass of about 1 amu.
Isotope: Atoms of the same element with the same atomic number, but different number of neutrons. Isotope of an element is defined by the sum of the number of protons and neutrons in its nucleus. Elements have more than one isotope with varying numbers of neutrons. For example, there are two common isotopes of carbon, 12C and 13C which have 6 and 7 neutrons respectively. The abundances of different isotopes of elements vary in nature depending on the source of materials. For relative abundances of isotopes in nature see reference on Atomic Weights and Isotopic Compositions.
Periodic Table Explorer 1.6 1.2
Atomic weight: Atomic weight values represent weighted average of the masses of all naturally occurring isotopes of an element. The values shown here are based on the IUPAC Commission determinations (Pure Appl. Chem. 73:667-683, 2001). The elements marked with an asterisk have no stable nuclides. For these elements the weight value shown represents the mass number of the longest-lived isotope of the element.
Electron configuration: The distribution of electrons according to the energy sublevels (subshells) in uncharged atoms. The noble gas shown in square brackets (e.g. [He]), marks that all the subshells associated with that element are fully occupied by electrons.
Principal energy level (Quantum number: n) | Sublevels available (Quantum number: l) |
1 2 3 4 5 6 | 1s 2s 2p 3s 3p 3d 4s 4p 4d 4f 5s 5p 5d 5f 5g 6s 6p 6d 6f 6g 6h |
Ionization energy (IE): The energy required to remove the outermost electron from an atom or a positive ion in its ground level. The table lists only the first IE in eV units. To convert to kJ/mol multiply by 96.4869. Reference: NIST Reference Table on Ground states and ionization energies for the neutral atoms. IE decreases going down a column of the periodic table, and increases from left to right in a row. Thus, alkali metals have the lowest IE in a period and Rare gases have the highest.
Other resources related to the Periodic Table
Periodic Table Explorer 1.6 4.6
- Chemical Evolution of the Universe